Why the Cost of Carbon Capture and Storage Remains Persistently High
The Bottom Line: Unpacking the future of Canada's oil & gas
Re-Energizing Canada is a multi-year IISD research project envisioning Canada's future beyond oil and gas. This policy brief is a part of The Bottom Line series, which digs into the complex questions that will shape Canada's place in future energy markets. (Download PDF)
Summary
-
Carbon capture and storage (CCS) costs depend on the process type, capture technology, carbon dioxide (CO2) transport, and storage location. CO2 capture costs are projected to range from CAD 27–48/tCO2 for processes with concentrated CO2 streams to CAD 50–150/tCO2 for diluted gas streams. The actual cost of CCS projects in Canada indicates that costs are in the upper range of what is predicted in the literature.
-
The persistent high costs of CCS are attributed to high design complexity and the need for customization that limits the deployment of CCS.
-
Comparing the experience rates—or the decrease in cost with increased development and deployment—of CCS with other energy technologies, such as solar and wind, shows that CCS cost reductions have been slow, despite being in use commercially for more than 50 years.
-
The economic viability of CCS for the oil and gas sector continues to rely heavily on federal and provincial government financial support. This is in contrast to renewable technologies, which have generally required government subsidies only in the initial development phases.
-
CCS may play an important role in hard-to-decarbonize industrial sectors such as cement and steel, where substitute materials or fully matured decarbonization technologies are not yet available or fully developed.
Carbon capture and storage (CCS) technology aims to reduce emissions by capturing carbon dioxide (CO2) and either burying it underground or utilizing it in other industrial processes. Unlike direct air capture and storage, which is a negative emissions technology that captures CO2 directly from the atmosphere, CCS captures CO2 from point sources, such as industrial facilities or fossil fuel power plants. Some CCS technologies have been commercially used for several decades and were initially developed for capturing CO2 from natural gas production for enhanced oil recovery—a process in which CO2 is injected into aging oil wells to increase oil production and extend the life of wells.
In Canada, there are seven commercial CCS projects currently operating—five in the oil and gas sector, one in coal-fired electricity generation, and one in the agricultural sector—capturing only 0.05% of national emissions. Despite the small number of operational projects and the limited efficacy of CCS in reducing emissions in Canada to date, Canada’s climate plan emphasizes the potential of CCS in the oil and gas sector to develop the technology and reduce emissions from production. The oil and gas sector is also foregrounding CCS as the primary solution for the sector to reduce emissions. The largest proposal has come from the Pathways Alliance, a coalition of Canada’s six largest oil sands producers, with a plan to build a CCS network in Alberta that proposes a combination of technologies to capture, transport, and store CO2 emissions from over 20 oil sands facilities. The coalition hopes to use this network to reduce 22 MT of CO2 by 2030. The Alliance has indicated that the CAD 16.5 billion project would be contingent on substantial government subsidies, noting the need for funding levels to be competitive with countries such as Norway that cover two-thirds of project costs and all operating costs for a decade.
Advocates for CCS implementation in Canada’s oil and gas sector envision it as a viable emissions reduction strategy that will see costs decrease with increased investment. However, the likelihood of substantial cost reductions remains uncertain. This brief explains the persistent high costs of CCS.
Calculating the Costs of CCS
Calculating the costs of CCS is complex, given the different approaches to measuring costs as well as varying applications of the technology and contexts in which it is used.
CCS technology requires significant inputs of energy to operate, and if that energy is provided by a fossil fuel, it produces emissions. There are two ways of measuring the costs of CCS per tonne of CO2 sequestered—CO2 captured and CO2 avoided—and only the latter accounts for the extra energy and emissions required to operate the CCS. CO2 captured refers to the total amount of CO2 collected by the CCS technology within a specific time frame, disregarding the extra emissions generated by the CCS process itself, whereas CO2 avoided is calculated by subtracting the increased CO2 emissions from the energy required to operate the CCS plant from the total CO2 captured (as illustrated in Figure 1). Therefore, the cost of CO2 avoided (per tonne of CO2) is the more appropriate measure to evaluate the cost of CCS, though estimates using this measure are limited and may only be available for some processes and sectors using CCS.
Figure 1. Two ways of measuring upstream emissions reductions from CCS for oil and gas: CO2 captured vs. CO2 avoided
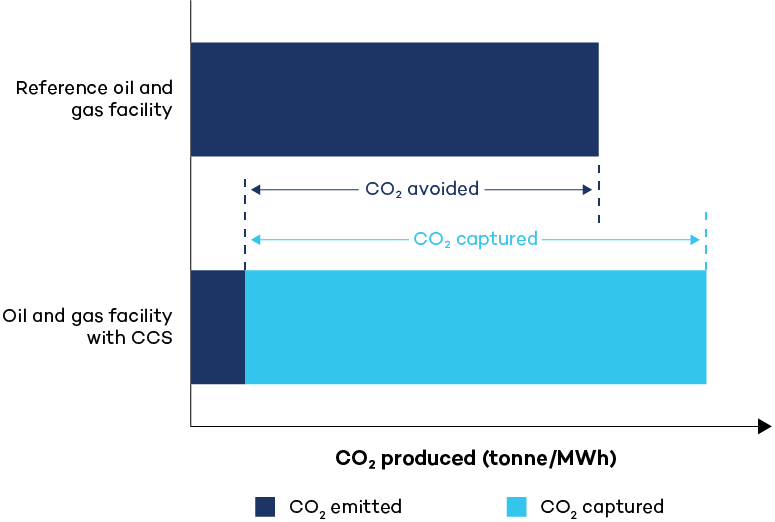
Source: Adapted from Wilberforce et al., 2021.
The costs of CCS technologies, as projected in the literature globally, vary significantly depending on the type of capture process employed, the means of CO2 transportation, and the storage location. Costs also vary depending on the CO2 concentration in the emissions stream: the lower the CO2 concentration in the gas, the higher the energy demand required for separating out the CO2, resulting in higher costs. Industrial applications like natural gas processing and ammonia production already have a high degree of CO2 concentration, leading to lower CCS costs. According to the literature on estimated costs for CCS in various industries, the cost of CCS processes with concentrated CO2 streams, such as from natural gas processing, ranges from CAD 27 to 48/tCO2 captured. By comparison, more diluted gas streams, such as coal-fired power plants, steel, cement, and some hydrogen production, are higher cost: cement production is estimated at CAD 50–150/tCO2 captured (CAD 45–205/tCO2 avoided), and coal-fired power plants range from CAD 26 to 173/tCO2 captured (Figure 2). These estimates do not include the added costs of transportation and storage. A recent study estimates a cost of CAD 111–144/t CO2 for a CCS retrofit on a natural gas-fired power plant at an oilsands facility.
Given the limited number of operational commercial CCS facilities, these cost estimates are predominantly based on modelling studies that employ various assumptions to forecast costs for theoretical facilities. This is a key reason for the broad variability in cost ranges, which may not accurately indicate the costs of actual projects.
Figure 2: Estimates of carbon capture costs by industry and category of capture technology (2021 CAD)
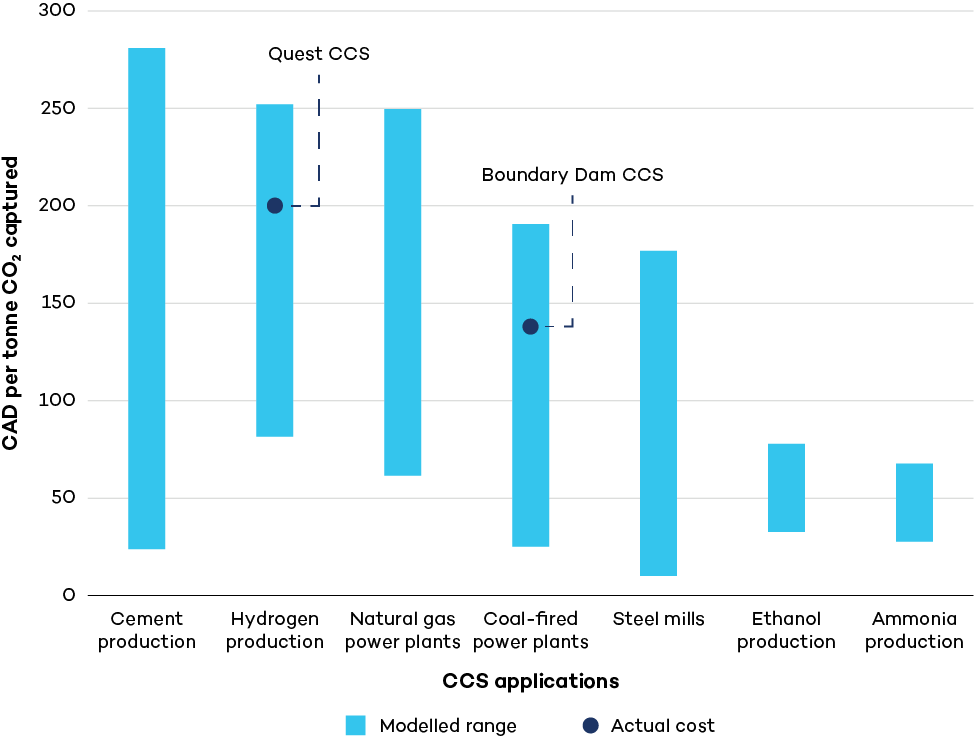
In the Canadian context, there is very limited data on the actual costs of CCS projects to date, but the few that are reported are at the higher end of the modelled cost ranges. For example, the Quest project, which captures CO2 for use in upgrading oil extracted from oil sands, costs around CAD 200/tCO2 up to 2021 (Globe and Mail Editorial Board, 2021). Meanwhile, the estimated lowest cost of capturing CO2 from the Boundary Dam project, a coal-fired power plant, is CAD 100–120/tCO2, but the project has repeatedly faced cost overruns and delays. CCS projects require a substantial initial investment, which is amortized over time. Thus, the eventual cost of CO2 captured and avoided is contingent upon the facility’s lifespan, production rate, and the effectiveness of permanent CO2 storage, all of which exhibit considerable variability.
The Persistent High Costs of CCS
The cost of CCS is currently high and varied, yet CCS proponents speculate that costs will decline as more investment drives innovation and learning. While this logic applies to many technologies, whether it applies to CCS is questionable due to its complex functional requirements and constraints.
Typically, the costs of a technology tend to increase during its initial phases, spanning from research and development to its demonstration. However, as the technology reaches commercial maturity, these costs often start to decline. This downward trend is captured through an experience rate, a metric commonly used to project how costs will reduce as a technology is more widely deployed.
CCS has a relatively low experience rate. This is due, in part, to characteristics inherent to CCS technology—including high design complexity and the high need for customization—which present obstacles to technological advancement. Design complexity refers to the large number of technical components in a technology and the extent to which they are interrelated. As in the case of CCS, high design complexity involves multiple interactions between the components, which makes technological innovation more difficult, leading to a highly iterative process with a high risk of bottlenecks and dead ends. CCS also has a high need for customization to specific applications, making it challenging to achieve large-scale deployment, limiting innovation acceleration, and, therefore, impeding cost reductions. While the overall process of CCS technologies is projected to be standardized, some components will need to be tailored to specific applications, geological conditions, and local supply chains, indicating a medium to high need for customization (Stephens & Jiusto, 2010).
Finally, CCS application in the oil and gas sector will have limited opportunities for learning by doing, since there are only a small number of operations in which it would be used. The specific applications of CCS in Canada’s oil and gas sector currently are hydrogen production for use in refineries and bitumen upgrading and one recent project using natural gas combustion. The three Canadian projects that capture carbon in hydrogen production are among the only commercial projects of this application in the world—the first of which came online less than a decade ago. Natural gas combustion applications of CCS are even more nascent, with the only existing commercial project coming into operation in 2022, even though some have indicated this would be the primary application of CCS in oil sands production. Although the Pathways Alliance coalition of oil sands producers has not disclosed details of the technologies to be used in their proposed CCS network, their announcement foregrounds “piloting next generation technologies” that are not yet commercially viable. Given that these technologies are in the early stages of development, and given the weak learning and experience rates exhibited in other CCS applications, the costs of CCS as applied in the oil sands may similarly fail to come down. This reality also prevents CCS in oil and gas from benefiting from the kinds of economies of scale that have accrued from the mass manufacturing of solar panels and wind turbines.
CCS for Oil and Gas Outcompeted by Renewable Energy
CCS is a technology to reduce greenhouse gas (GHG) emissions, and so it must be judged against other such technologies, including the use of solar photovoltaic (PV) and wind turbines to lower emissions by substituting for gas- and coal-fired electricity generation. Compared to solar and wind, CCS has had a low experience rate (Figure 3). Reported experience rates for CCS used with a natural gas combined-cycle plant range from 2% to 7%, compared to a 23% median experience rate for solar PV since 1976. This means that the cost of solar PV modules decreases by 23% every time the global installed capacity doubles.
Figure 3. Experience rates for various technologies globally
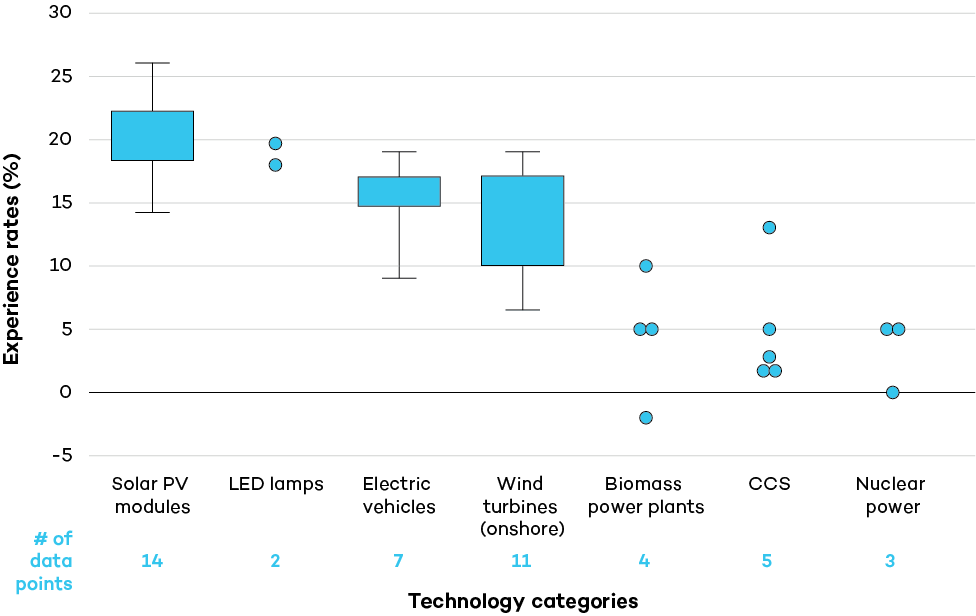
Source: Adapted from Malhotra & Schmidt, 2020.
As these experience rates suggest, renewable energy technologies have undergone significant cost reductions in recent years. This trend makes the financial viability of CCS deployment, particularly in the electricity production sector where it directly competes with renewables, increasingly questionable. Solar PV and wind power are notable examples of renewable technologies that have been developed more successfully. These renewable sources have generated revenue through electricity sales, enabling them to achieve cost competitiveness through increased deployment.
While CCS is likely to be outcompeted in the energy industry, its potential use in other sectors should be evaluated separately, particularly for hard-to-abate industries such as steel and cement that have limited alternatives for emissions reductions. Notably, the Intergovernmental Panel on Climate Change identifies CCS as a vital mitigation tool in the cement industry, given that two thirds of emissions from cement production stem from chemical reactions when heating limestone. In other cases, alternative decarbonization options may be more efficient and cost-effective, such as scrap steel recycling or the use of green hydrogen to produce direct-reduced iron in the steel industry. Due to its high costs and the complexity of the technology, CCS should be reserved for challenging industrial processes, such as those involving carbon-intensive chemical reactions and high-heat processes, where electrification and other decarbonization alternatives are not readily available. More research and development are needed to confirm whether the technology can be made effective and competitive in these sectors.
CCS in Oil and Gas Is Expensive, and Public Investment Is Better Directed at Cost-Effective Solutions
In contrast to technologies such as solar PV and wind that require subsidies only initially as the technologies scale up, CCS in oil and gas production requires substantial, ongoing government support and regulations. To date, in Canada, stubbornly high costs of CCS in the oil and gas sector have been offset by extensive government subsidies and tax advantages. For example, CCS projects in Alberta—the country’s largest oil and gas-producing province, where most CCS projects are proposed—are eligible for credits under the provincial Technology Innovation and Emissions Reduction regulations and may also be eligible for support from the new federal CCS investment tax credit and clean fuel regulations. While oil and gas sector representatives argue that additional public funding is needed for CCS, independent analysis reports that these and other significant credits are sufficient and are indeed more generous than American CCS incentives under the Inflation Reduction Act.
The pursuit of CCS in the oil and gas sector should involve weighing CCS against all other options for reducing GHG emissions. Given the relatively high costs of CCS per tonne of GHG emissions reduced, CCS for oil and gas is an inefficient and risky use of public funds. Faster, more effective options for significant GHG mitigation in the sector—such as the reduction of methane emissions, electrification, and efficiency measures—should be pursued by the industry.
It is not likely that the costs of CCS in Canada’s oil and gas sector will decline significantly over time: the technology is too complex, it demands too much customization with each application, and it is unlikely to capture the benefits of mass manufacturing in the way technologies like solar PV have. Industry calls for additional public support for CCS should be closely evaluated to ensure any public dollars are directed to sectors that have a viable 1.5°C trajectory. The oil and gas sector has yet to demonstrate that CCS can achieve this.
A full list of references can be found here.
Re-Energizing Canada is a multi-year IISD research project envisioning Canada's future beyond oil and gas. This publication is a part of The Bottom Line policy brief series, which digs into the complex questions that will shape Canada's place in future energy markets.
You might also be interested in
Powering the Clean Energy Transition: Net-Zero electricity in Canada
This brief explains how a shift to clean power generation can offer affordable, reliable electricity, benefiting households and businesses alike.
Why Liquefied Natural Gas Expansion in Canada Is Not Worth the Risk
An analysis of the economic and environmental risks of liquified natural gas expansion in Canada.
New Report Highlights Economic and Environmental Costs of Canada’s LNG Expansion
New report explains how LNG expansion will not only hamper Canada’s progress toward its climate goals but also create challenges for the economy in the long term.
Fueling Change: The journey to end fossil fuel subsidies in Canada
How Canada became the first country in the world to introduce a framework for ending government subsidies to domestic oil and gas companies.